Clunky and overpriced?
Not in 1984, when consumers lined up in droves to buy the first cellular
phone as soon as it hit the market. And certainly not to Rudy Krolopp, lead
designer of the Motorola DynaTAC 8000X.
Krolopp, now 74 and retired, still gets a "warm fuzzy feeling" thinking about
the DynaTAC and knowing that "a handful of us did something that was really
significant."
This brick took over a decade to get to market.
Krolopp was assigned the project by Martin Cooper, who ran Motorola's
research and development effort in wireless and was ultimately dubbed the father
of the cell phone by then-CEO Robert Galvin. Both Cooper's and Krolopp's names
are on the original patent along with that of John Mitchell, former head of the
company's communications division.
"Marty called me to his office one day in December 1972 and said, 'We've got
to build a portable cell phone,"' Krolopp recalled. "And I said 'What the hell's
a portable cell phone?"'
Talk about short talk time -- Krolopp and his team were given six weeks to
come up with a working model.
The urgency was because the Federal Communications Commission (FCC) was
deliberating over whether to allow AT&T to set up a network to provide wireless
service in local markets, and the phone company itself was considering making
wireless phones. Motorola didn't want to be shut out.
After the meeting with Cooper, Krolopp got together with his design staff and
built a conceptual model.
"We sprung it on the engineers one day," he said, recalling their surprise at
how small it was at the time. "There were only eight guys in the room. Cooper
said 'Anybody who doesn't believe this can be done, leave the room."'
No one did. But it was another 10 years and a total of $100 million in
development costs before the phone was officially unveiled in 1983 -- the delay
resulting mostly from the need to build towers and infrastructure.
Krolopp says Motorola's new Razr is "really a cut above" anything that was
done in the past but still represents only "the tip of the iceberg" of what
phone designers will be able to do.
"Oh, would we have loved to do that back then," he said of the Razr's
wafer-thin size. "We had the capability to design it but we didn't have the
capability to build it. We couldn't get batteries down that small, couldn't get
antennas that small, couldn't get key pads to work that way.
"Technology has changed so much."
2005/04/25
(AP) -- Jay Leventhal, who is blind, still
fumbles with the tiny controls on his iPod but has given up on the kiosk in his
New York office building that lists all the tenants.
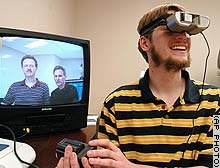 |
A set of goggles called JORDY functions like two
high-definition television sets, with controls over color, contrast and
magnification. |
For Leventhal, even laundry has become a task requiring the help of a sighted
person. The washers he uses now takes smart cards instead of quarters, issuing
instructions on a digital screen that he can't read.
As technology has evolved, it's become lighter, smaller and more portable.
For most people, that makes it more convenient. For millions of blind and
vision-impaired people, it's anything but.
"The biggest barrier for blind people is access to information, and more and
more information is being made available through different machines that aren't
designed for people who can't see," says Leventhal, editor in chief of
AccessWorld: Technology and People with Visual Impairments.
Blind people need a way to communicate with the machines that surround them,
he says, from automated tellers to ticketing machines at train stations and
airports.
Leventhal and other experts on assistive technology say there's no reason
that can't happen. The technology exists in voice chips, image processors, cell
phones, cameras and personal digital assistants.
Someone just needs to put it all together.
That's the principle behind the Levar Burton Vision Enhancement Technology
Center, a fledgling venture in Morgantown, West Virginia, that will pair the
resources of West Virginia University and Georgia Tech with private-sector
partners like Motorola Corp.
Levar Burton, who played blind Lt. Geordi La Forge in "Star Trek: The Next
Generation," is lending his name and star power to fund-raising efforts for the
center.
Though he's not blind, he wore a visor on the set that impaired his vision by
75 percent for nearly 12 hours a day.
The center and its partners will use off-the-shelf technologies like lasers,
magnifiers and global positioning systems to develop, test and market products
to help people see better. The American Foundation for the Blind, which runs a
technology evaluation center in Huntington, West Virginia, will advise the
scientists.
Of the 18 million Americans with diabetes, for example, about 5 million are
visually impaired.
But when Mark Uslan, director of the Huntington facility and his lab
volunteers tested 30 brands of blood glucose monitors, they found only one that
was usable -- but it was 10 times larger and 10 times more expensive than the
other models tested.
Mainstream companies need to consider the vision-impaired when designing
products, Leventhal says.
"There's no reason for someone to have to make an MP3 player that's
accessible to blind people when several companies are already making MP3
players," he says.
Though many assistive devices are commercially available for the blind and
vision-impaired, each has limitations and nearly all are expensive, produced in
small batches by specialized companies. Even a software program that makes a
computer talk is nearly $1,000 -- as much as the computer itself.
And with few health insurers willing to pay, sales are too small to justify
significant corporate investment.
"That's why we've had to take this avenue," says Dr. Richard "Scott" Hearing,
director of the Low Vision Clinic at Jupiter Eye Center in Florida and an
adjunct faculty member at WVU. "If there were a lot of money to be made in this,
someone would have already done it. ... It's not the cost of the technology
that's expensive; it's the cost of adapting it for vision impairment."
A few companies are working on assistive technology, but one of the largest
and oldest, Telesensory Corp. of Sunnyvale, California, went bankrupt and closed
last month.
Jody Ianuzzi, program coordinator at a blindness training center in Florida,
says cost is critical. Some people will find state programs to pay for devices,
and others have employers who will buy them as a reasonable workplace
accommodation. But for retirees and the under- or unemployed, she says, "one
device could break the bank."
Hal Reisiger, president of Enhanced Vision Systems of Huntington Beach,
California, says that's why his firm will partner with the Levar Burton Center;
new products must be practical for the manufacturer, too.
"We could make flying saucers," he says, "but if people can't afford it, it's
not an effective mode of transportation."
Hearing and others aim to keep costs low by designing not only assistive
devices but also mainstream products with military and recreational
applications.
Burton's Star Trek character is the inspiration for one of the most advanced
devices on the market today, a set of goggles called JORDY, or Joint Optical
Reflective Display.
It functions like two high-definition television sets, with controls over
color, contrast and magnification.
But the JORDY is heavy, offers a limited field of view and lacks image
stabilization, so it can cause motion sickness. And it costs about $3,000.
Paul Mogan, a legally blind electronic engineer at NASA's Kennedy Space
Center in Florida, says JORDY is best suited to stationary tasks like reading.
He wants to help create the next incarnation, special sunglasses linked to a
wireless computer that can fit on a belt or in a pocket.
With a voice chip, GPS and image processors, the visor could serve as a sort
of on-board navigation system for the blind, calling out hazards, announcing
nearby shops, even reading signs that say what's on sale.
NASA has a compatible goal: The space agency wants a wearable wireless
computer that would help technicians work independently outside a spacecraft.
"NASA has this initiative to go to the moon and Mars, and you're not going to
be able to take a ton of crew, so you're going to have to be very efficient in
what you're going to do," Mogan says. "All people have to be able to have access
to a lot of information."
2005/05/05
ConnectPress's COFES Contest Runner-Up: Innovations
That Will Make Your Engineering Life Easier
By Michael Lynch, CEO, Right Hemisphere
By Kim Spinsby
Predicting innovative trends we will see in the next 2-5 years in engineering
and manufacturing is an easy task. Most PLM developers are already busy coding
the offerings their users will see in two years, and the foundations and
architecture are already formed for the next five years.
Several emerging technologies and trends - just beyond the 5 year horizon - are
already visible. Others are already viable, and suggest not only improvements to
our current tools and processes for design, but major shifts in the way we
define and practice design and engineering.
Imagine designing unrestricted to an office, or even a monitor or keyboard, and
with all desired information, without excess or overload. Mundane and repetitive
tasks will be minimized and true productivity will increase. The only roadblock
will be the lagging organizational acceptance of change in social systems and
management attitudes. More on this later.
Engineers and their companies are tasked with offering solutions that both solve
a specific set of needs and give unique advantages to the consumer. Rarely does
this come in the form of radically new concepts. Most of our products come from
stretching the known characteristics: something that is larger, smaller, faster,
more precise... or that has other performance improvement, price differential,
better aesthetics or other bells and whistles. With this understanding, let's
take a brief look at trends and innovations.
TREND: Creation Enabler vs. Creator
As we attempt to "design" new solutions with our current suite of tools, we
continually search for new processes or functionality that would make us more
efficient in completing the path from need to a "product" or solution. Engineers
typically are first, collectors and organizers of information, building an
information mountain. As we start to form initial concepts to meet requirements,
and then redefine them, we find information and its re-use becomes the driving
means of productivity. Then we become sculptors, evaluating and discarding all
unneeded data, until the remaining solution is simple and elegant.
Observing millennia of history, and several decades personally, I have seen a
common trend: what we take for granted today was once a luxury or a skill of a
specialist - a "black art" in its day of conception. We engineers are today’s
specialists. In the future, the data we collect now will already be collated,
configured to allow the consumer to become the product engineer.
The true inventor/designer of all products and services is the consumer. His or
her need drives our developments and success. I’ve watched the CAx market
develop from the days of computer programmers drawing circles on storage tubes
and expect the future to allow consumers to create products by combining
characteristics to meet their unique sets of needs.
Engineering in the future will be defined as the process that enables anyone to
become the actual designer of all products and processes. It will truly be the
customer who intends to use these products or processes. The role of the
engineer will change from being a creator to an enabler of the consumer's direct
creation. Anyone will be able to quickly pull characteristics from multiple
solution components and watch incredible computing power link virtual modular
scenarios into unique custom products. Everyone will be using a continually
learning, collective intelligence, based on the foundations of AI.
TREND: Moving the User From Proximity to the Computing
Core
My personal experience the past few years has been with mostly metal fabricated
structures and assemblies, constructed from joining components of formed metals.
Initial design constraints are defined by the customers: functions, performance,
physical characteristics (size, weight), environment.... Most engineers
initially draw from accepted past exercises and experiences, only stretching the
current to accommodate the expanded needs. Some of the most practical
"innovations" come from observing a desired phenomenon in an unrelated simile,
such as applying a concept from cosmetics into an industrial coating - aka
"paint."
Since most innovation is often a recombination of modules, AI principles would
suggest that the first choice would use qualities and features in a slight
variation of an existing combination.
When a designer has chosen a solution (component) for evaluative iteration, and
decides to include it in an assembly (utilize electrical characteristics), or
package the solution, he needs his chosen characteristics to become quickly
available for evaluation.
Envision a suite of tools that transcends all enterprise operations, helping
engineers, managers and all members of their companies to offer unique
solutions, serving the desire of corporate and individual consumers. Though this
is a lofty goal; we must start in the mind of the engineer.
Example: A switch in an assembly. Electrical factors: configuration (spst),
performance (amps, voltage, load). Mechanical factors: size, how mounted,
environment. If the switch needs to be assembled into a larger assembly, how
will it be mounted? Does it come with mounting holes? If yes, this suggests that
it will be attached. Then what are the size of the holes, and thickness of the
feet? They assume a suggested mounting and that the holes would be standard
hardware choices.
What connections need to be made? And what connection conditions are required?
Example: A proposed electrical product. Defined by a function (circuit), and a
package. Our mechanical designer starts with a proposed circuit (an electrical
assembly) and then attempts to choose the components to satisfy the circuit
designer's intent. He queries all known sources available as potential
innovations, but weights choices based on past experience of the company or the
market. As the specifications are added, the choices are quickly narrowed.
What we need is a process to collect all available solutions, but weight their
selection by current experience, use or known failure characteristics.
Ultimately, a search engine would browse and pre-select all available choices,
all available information; then selectively narrow choices and track the
importance of which characteristics were used to narrow the selection. This data
would be used to optimize resources in future selections.
At the same time, the perpetual search should be continued, collected, and
indexed to meet future needs not yet defined. Any resource idled needs to be
sensed and utilized to continue expanding the search and reducing duplicated
data and solutions (product differentiation and market definition). Finally, the
system should anticipate a resource’s use the next time a similar association is
needed – by recognizing a pattern of use.
TREND: Creation Engines vs. Requirements Management
I learned early in my life, as a pilot and engineer, several key concepts. Like
life, and business, engineering is a negotiation of compromises. It is:
1) The most acceptable solution currently available, constructed from a process
of combining a balance of conflicting needs, by selecting desired traits.
2) There is no such animal as designing a solution that will never fail. Instead
we design a solution whose failure is beyond the specified requirements and
fails in an expected, predictable manner, avoiding undesired consequences.
A good example of a design process that can be optimized is the process of
making an assembly of components. The manufacturer will provide all the
characteristics in a representation that allows its virtual inclusion by a
designer into an assembly. Initially, this means in a "lightweight,"
vendor-neutral format that allows a designer to utilize the "solution" in
his/her concept. Since I work in an industry that has many different "designers"
- electrical, mechanical and "business" - each has a subset of the information
they deem important to their role in the design and manufacturing teams.
An electrical designer desires the "solution" (component) to meet performance
characteristics. The mechanical designer for the same "solution” (product) needs
to meet many physical characteristics as well as connectivity. Since both are
"looking" for solutions, they must be able to quickly evaluate a world of
potential options and extract their needed piece of data, quickly and
efficiently. A solution is currently available through the information delivery
of the internet, and selection is aided through "search tools." The first time,
this is an effort-consuming task for a designer.
To extract the needed characteristics from an almost infinite offering without
being drowned in unneeded information is a challenge. The process translates
into what is currently known as requirements management. If we combine this with
a learning, collective set of rules (AI), we can optimize the search and
selection process, to optimize a very valuable resource... time.
But creativity may find a better solution which falls OUTSIDE the limits of
rules we build. What we need is a constant automatic analysis of solutions not
chosen, in potentially unique combinations not previously considered. Some
simple experiments of this have been undertaken by "creation engines," which
automatically iterate potential combinations that would be initially eliminated
by rules sections based on previous experience (in the box).
Hardware/Interface Trends
Here I offer a few observations and opinions about how we will relate to our
computers in the future.
TREND: Input and Output Devices Getting Smaller, Lighter,
Lower or Empowered - and "Further from the Computing Core"
Current: Viewing and projection devices tied to a physical computer.
Future: Common use of projection (heads up) and wireless, lightweight portable
(PDA-like) display devices, integrated with eye-tracking and command selection
and acceptance devices. Wearable/portable computing takes off via these wireless
interface (I/O) devices, further separating users from core computing power,
releasing designers from keyboard, mice, desks, and offices.
Current: Spaceball and mice.
Future: Body-mounted, multi-axial wireless devices (6 degrees of freedom (DOF));
a wireless "ball" that you move in three axes, turn in your hand, bring closer
or further (zoom), 6 DOF. It would be tied to a 3-dimensional tracking device,
measuring all six of its six DOF. This would preferably be passive (unpowered),
maybe transponder-based. And later, possibly the wireless ball has the image
projected inside it.
These could be teamed with a pair of active image opticals (primitive=glasses)
that can be turned to any point of view, zoomed, cut and layered. They would
include attributes that can be summoned and queried at will and include
eye-tracking and command acceptance devices.
The next wave would include a PDA-like display device that has a pop-up screen
to enable holographic display coupled with keyboards. This would then give way
to portable and lightweight wireless "chording devices," combined with armature
suits; and/or non-invasive myelosensitive interface wristbands that translate
small, non-stressed body movements to "input."
Speech Technologies as input: I also see what I once discarded - voice input and
searches - as becoming viable and the preferred input device for many
transactions. In the past, the thoughts of designers sitting adjacent in shared
cubicles, calling out verbal commands to their workstation was horrifying. But
as speech technology progresses, starting with the use of "dictation cups" and
voice recognition, and continuing with increasing use of AI to anticipate and
evaluate functions, this will become more viable.
Process Trends
Trend: Lightweight, "streaming" graphics models can be displayed quickly in
simple form (lightweight data), automatically adding anticipated layers of
detail or manually providing requested detail.
Trend: Bandwidth increases and the team disperses (elimination of the "team
workplace"). As bandwidth increases, I see a general trend for the central "team
workplace" to be dispersed. Also, it will become significantly more profitable
for design teams to require continuous physical presence (proximity) to other
members of the team. Better virtual communication technologies will accelerate
this, along with commonly accepted productivity metrics of the design and
engineering processes, tied with better methods of tracking competencies.
Reward/pay will truly be based on contribution and its measured metrics. It will
not be dependent on where the team member chooses to work, but on the
significance of the contributions.
A few other innovations I’d like to see:
1) A combined Google and Thomas Registry-like product would search
internationally accepted (uniform) standards for describing components or
products, specifically for reusable components.
2A) A partial data input or "scan" would compare a physical object by perceived
characteristics, and, through AI pattern recognition, would identify the
"object" and add it to a collective worldwide database.
2B) An analytical chamber for scanning objects for shape, density and many other
functions would have multiple (non-destructive) capabilities, and apply chosen
analysis methods based on physical characteristics (super CMM) with X-ray,
diffraction, resonance, imaging... for reverse engineering.
3) Bi-directional data sharing between design organizations would allow a
collective, neutral, and user-anonymous database. It would be generically
encoded and possibly identification key-coded, and collection of the inquiries
would be compiled for the sake of accuracy and use of all. Proprietary data
would be protected. Anyone designing would get interactive suggestions based on
the experience of all, including generalized costs based on batch size, tooling
costs, shipping information including packaging and transportation costs, and
regulatory constraints, including recycling costs and procedures.
Initially, some of the emerging technologies mentioned in this essay will be
disruptive. The most probable and successful early adopters will be those
unencumbered by a legacy of forcing new tools to implement or mimic old
processes. In a short time, many of these innovations will free us from
technical and social restraints, enhancing our personal and collective
creativity, and vastly improving productivity. The ultimate effect will be the
engineer creating the tools to enable consumers and customers to become the
creators.
2005/06/04
Rapid Injection Molding Brings New Possibilities
By Brad Cleveland, president and CEO, The Protomold Company,
Inc.
Now more than ever, technological advancements drive the product design
process. Increasingly powerful CAD programs allow more complex product designs,
which in turn drive the demand for more complex prototypes. At the same time,
fast-moving competitive markets require frequent design changes, shorter
lead-times, and tighter budgets. In short, prototyping must be faster, better,
and less expensive.
While traditional rapid prototyping is still widely used, a growing number of
design engineers are turning to rapid injection molding for prototype
development. Pioneered by The Protomold Company of Maple Plain MN, rapid
injection molding is based on the use of proprietary software technology that
automates the process of designing injection molds and a streamlined mold
manufacturing process, resulting in fully functional parts while dramatically
reducing the time and cost normally associated with conventional injection
molding. As acceptance grows, rapid injection molding is changing the way
designers think about prototyping in industries like aerospace, appliances,
automobiles, electronics, and medical devices.
Different Processes for Different Needs
While rapid prototyping and rapid injection molding both start with a 3-D CAD
part model, the actual processes and end results are very different. Rapid
prototyping, which includes technologies like stereolithography, selective laser
sintering, fused deposition modeling, laminated object manufacturing and three
dimensional printing, creates a prototype layer-by-layer to form the end
product.(1) Rapid injection molding, on the other hand, uses the familiar
process of injecting heated thermoplastics into a metal mold, where the material
cools into the desired shape.(2) Unlike rapid prototyping, rapid injection
molding produces a fully functional, injection-molded part. The resulting
quality difference is so significant that many design engineers who test form
and fit using rapid prototyping will still check the functionality of their
prototypes using rapid injection molding. Also, while conventional mold-making
is very labor intensive, rapid injection molding fully automates this step,
typically reducing tooling cost and lead time by two-thirds.
Quantity and Quality Considerations
Rapid prototyping may be an acceptable choice for creating small numbers of
prototypes in very short lead times – typically fewer than 10 parts in one to
five days. But rapid injection molding can economically deliver 25 to 1,000
production-quality prototypes in 3 to 15 days. When production-quality surface
finishes are not required, rapid prototyping may be an acceptable choice, but
the “stairstep” surface it leaves on parts is a significant disadvantage, as it
keeps parts from fully reproducing the intended design. Rapid injection molding,
on the other hand, uses a CNC-machined metal mold to create the part shape, so
it can replicate the intended shape much more accurately, just as with
conventional injection molded parts. This can greatly increase the value of the
prototype to the design engineer.
Furthermore, rapid prototyping is very limited with respect to materials that
can be used and often creates prototypes too fragile for rigorous physical
testing. Rapid injection molding can utilize a wide range of production-grade
resins to manufacture fully functional parts, allowing the design engineer to
consider both mechanical properties (e.g. strength, temperature resistance,
etc.) and cost when ordering the prototypes. At Protomold, customers can select
from hundreds of in-stock resins or provide the material themselves.
Rapid Injection Molding for Design, Testing and Production
Rapid injection molding can be utilized from the earliest stages of product
development through bridge tooling and ongoing production. After rapid injection
molding is used to create prototypes, it can support the manufacture of 1,000,
even 10,000 parts for pilot production or market testing at no additional
tooling cost. If high production volumes do justify production steel tooling,
rapid injection molding can be used as bridge tooling, to produce fully
functional production parts until the steel tool is available. And when
production volumes are moderate, rapid injection molding can be a complete,
cost-effective production solution.
How Rapid Injection Molding Works
Rapid injection molding is a unique, highly automated method of producing
injection molded parts from a 3-D CAD part model. The core technology is
proprietary software that automatically converts the part model into toolpaths
for CNC milling machines. These in turn produce the mold components that, when
assembled and mounted on an injection molding press, produce the desired part.
(See
Figures 1 and 2. Open the image window above and click on the Forward button
to scroll through images).
Although a vast array of geometries can be produced, some limitations in part
size and complexity exist due to the highly automated nature of the mold making
process. For example, CNC milling results in rounded external part corners. Some
high-aspect ratio features, e.g. thin ribs, may not be machinable. And the
ability to produce “undercut” part geometry features is limited.
However, adapting part designs to be compatible with rapid injection molding can
be very simple. After submitting a 3-D CAD part design via Protomold’s Web site,
design engineers receive feedback on part geometry and pricing via ProtoQuote®,
Protomold’s Web-based interactive software. ProtoQuote allows designers to
change parameters such as the number of cavities, A- and B-side finish levels,
and resin, as well as the desired delivery schedule. These changes automatically
update the price quote.
The software also provides a rapid injection molding compatibility review, often
suggesting changes that will improve moldability or reduce tooling cost. This
automatic analysis highlights undercuts, wall thicknesses that could cause fill
or sink problems, and areas where draft is required. The system also provides
color-coded indications of radii resulting from the mold-milling process and
areas where a minimum thickness might be required. If design modifications are
indicated, design engineers can consult Protomold’s online design guide or
contact the company’s engineering professionals for assistance.
When the order is finalized, the Protomold software automatically generates the
mold design, including core and cavity geometries, shutoff surface generation,
gate-design layout, and ejector-pin placement. The software then outputs
toolpaths to CNC machine cells to manufacture the required mold components.
Discovering the Benefits of Rapid Injection Molding
Trapeze Networks™, a wireless local area networking (WLAN) startup company based
in Pleasanton, Calif., recently collaborated with its design firm and Protomold
to manufacture a wide range of parts used in the company’s new WLAN Mobility
System™ product line. This suite of products shares a system-wide control plane
and data plane that delivers secure user mobility, seamless wired and wireless
integration, and tools to plan and manage large enterprises — before and after
deployment.
The company’s initial plan was to consider a two-step approach to product
development. In the first step, the company intended to use “rapid prototypes”
to verify form and fit. In the second phase, once the design had been refined
and stabilized, it would use a conventional injection-molding process to get
working parts and premium-quality prototypes to test functionality. But, on
advice of its design-engineering firm, Santa Ana, Calif.-based designsUNLIMITED,
Trapeze Networks decided to try an alternative process, using the relatively new
technology of rapid injection molding.
Because Trapeze received its rapid injection molded parts in just five days, the
company was able to skip the rapid prototyping step of new product design for
its Mobility Points™ product and move directly to functional testing and
production ramp-up — faster and more cost-effectively than they originally
believed possible.
Once the initial testing was completed, Trapeze proceeded with placing a
high-volume production tooling order from a current supplier and was quoted an
eight-week lead time. After showing this supplier what Protomold accomplished in
one week, the company was able to renegotiate the production-tooling lead time
to four weeks. During the interim from low-volume to high production, Trapeze
was able to use parts made from Protomold to support its production ramp-up
while the high-volume tooling was manufactured.
With the ability to obtain accurate, rapid injection molded parts, Trapeze has
radically changed its product development cycle.
New Possibilities
Before rapid injection molding, design engineers faced a large gap between the
capabilities of rapid prototyping and conventional injection molding. Rapid
prototyping parts were fast and relatively cheap, but weren’t “real”. Injection
molded prototypes were real parts, but neither cheap nor fast. With rapid
injection molding it is now possible to get real parts, real fast and at a real
savings. To find out more about rapid injection molding, visit The Protomold
Company’s Web site at www.protomold.com.
References
1. Worldwide Guide to Rapid Prototyping,
http://home.att.net/~castleisland.
2. Injection Plastic Molding Design Guide,
www.engineersedge.com/injection_molding.htm.
2005/06/29
Are We Asking the Right Questions About 3D Viewer
Technology? A Higher-Level View of Enterprise Data Assets
The global economy imposes ever-increasing pressures on individual companies.
The needs to control manufacturing costs and improve productivity are more
critical than ever for success. Manufacturing processes get more difficult
outsourcing and partnering introduce multiple levels of complexity for
operations management. Efforts to better leverage outside resources have
executives trying to find standardized technology and tools, especially when it
comes to sharing and accessing vital engineering data assets. The world of
digital assets has resulted in the emergence of impressive but confusing choices
for critical tools such as 3D viewers.
Emerging technologies invariably follow similar evolutionary patterns.
Initially, a breakthrough in one area leads to a variety of competing
alternatives, all claiming to be the best. For example, think back to the early
days of corporate computing. Several major computer vendors and lots of smaller
providers tried to convince potential customers that it was best to select one
computing vendor for all of their needs — One vendor with one system
architecture, one family of proprietary software solutions, and one support
organization that could handle all situations. But the customers soon discovered
the truth: choice was not about the selection of a single vendor. Instead, open
heterogeneous computing models were adopted as a means to integrate
heterogeneous computer platforms within their own organizations, and as a means
of communicating with partners and suppliers more effectively.

“Digital data assets remain virtually locked up within
separate engineering teams unless an enterprise can create a practical 3D asset
pipeline for reaching all of the various downstream users in manufacturing,
marketing, sales, and support.”

Today, the providers of 3D viewing technology would have us believe that we are
faced with a similar need to select a single, superior solution that can meet
all needs now and into the future. We can apply what history has taught us,
consider what we see happening, and make some educated predictions. In the
not-so-distant future, all of the 3D viewing products will provide similar
visualization, zoom, pan, annotations, measurements, and other capabilities —
all features being demanded by CAD users today. So too will the CAD formats
become less and less differentiated, yet still proprietary with respect to each
vendor’s viewer application. The 3D viewers and CAD formats will evolve to excel
in specialized tasks, to target specific industries, or to set themselves apart
in a variety of other ways. Engineering teams will be able to choose the viewers
that fit their projects, processes, and user needs. In fact, we are very close
to this reality today.
Solving the Real Problem: Adopting an Enterprise-Wide 3D
Data Model
What challenges are raised if we avoid the trap of standardizing on a single
viewer and its related 3D data format? Engineering organizations today are often
geographically dispersed, and multiple viewers are typical within the same
company. Outsourced suppliers and partners complicate the situation, inevitably
bringing additional 3D formats and tools into the picture. Even if companies
succeed at standardizing formats and tools in house, supporting all of the
various viewers and 3D data formats poses challenges when working with
outsourced suppliers and partners. More serious challenges exist for
non-technical users outside of engineering. Digital data assets remain virtually
locked up within separate engineering teams unless an enterprise can create a
practical 3D asset pipeline for reaching all of the various downstream users in
manufacturing, marketing, sales, and support.
Is it possible to create an enterprise 3D data framework that supports numerous
3D data formats and a hodge-podge of non-integrated tools? Digital data
management solutions do in fact exist, and can enable all-encompassing 3D data
strategies.
Shifting focus from 3D data formats to a 3D data strategy allows digital assets
to become an integral part of a product lifecycle management (PLM) strategy. The
enterprise 3D data strategy can address engineering visualization needs, and
also provide better returns on investments by enabling the application of
engineering data throughout the corporate infrastructure.
What should this 3D data strategy look like? Consider the napkin-to-retirement
lifespan of any 3D data asset and the many uses for the asset during that
period. Many collaborating design teams need the ability to share access to 3D
data, and non-engineering organizations would like to use graphics generated by
extracting and transforming 3D engineering data into formats that make sense to
their team (see Figure 1). By creating a product graphics management (PGM)
pipeline, enterprises could gain an open, scalable foundation. A broad range of
data formats, authoring tools, and third-party publishing applications could
coexist to facilitate the 2D and 3D graphics authoring and publishing process.